DC to DC converter design – Don’t blow your fuse (and other lessons)
on LinkedIn:
With a vast range of off-the-shelf and feature-rich control ICs available, the selection and design of DC to DC converters is a superficially simple process.
But even with these highly integrated parts and design resources there are still many common pitfalls that can lead to Electromagnetic Compatibility (EMC) or thermal nightmares, blowing budgets, timescales, and the occasional fuse in the process.
I’ll share a few of the most common “gotchas” that electronics engineers will contend with early in their careers. We’ll dodge the complex calculations here, but there are excellent resources such as “The Art of Electronics” that can provide a deeper mathematical explanation of the issues at hand.
Medical device or arc furnace? Context is key
You wouldn’t use a trailer truck to commute to work, nor would you use the family car to ship potatoes to a supermarket. Both are vehicles but, clearly, they operate in different contexts so have different strengths and weaknesses.
The same logic applies to switch-mode converters and controllers. A quick glance at the cover page of any switcher datasheet will often reveal potential use cases. This is not just marketing: if a switcher is intended for industrial machinery or large server farms, it is quite likely that its EMC performance faces different limits to those marketed at consumer or healthcare devices. For example, while higher noise limits in industrial settings liberate chip designers to focus more on power efficiency by maximizing switching speed and slew rate, this can also increase emissions.
Excessive switcher noise can lead to poor performance of analogue circuitry, and in severe cases can interfere with the function of nearby devices. So, while those efficiency figures might be tempting, using the switcher in a compact medical device could lead to months of work to achieve compliance in EMC test chambers.
Lesson: Use parts in the context for which they are designed. Parts targeted at industrial applications may lead to issues if used in consumer or medical devices.
The heat must go somewhere
So, you’ve found a very neat five-amp switcher with a 3x3mm footprint that is 90% efficient? Sounds perfect for your space-constrained, high-power design. Except you’ve just plugged it in, and it cuts out after ten seconds at load. This is less than ideal. The problem is that, even at 90% efficiency, the switching losses at 5A are enough to make a 3×3 chip quite toasty – so much so that the thermal cut-out is operating. You might have been fine if you had a large copper flood to take the heat away, but this is a space constrained design so of course you don’t.
Lesson: Always consider the thermal design of a high-power system. Even if a small switcher is electrically capable of high currents, it may require substantial heatsinking that wipes out any size or efficiency benefits.
Evaluation modules are there to help
Datasheets are an important resource for correctly integrating a switcher design into a larger electronic system, but they are also a company’s marketing collateral, and can mask certain “gotchas” in product performance.
They do not guarantee that your design or use-case will play nicely with the chosen parts. Evaluation modules (EVMs) provide a less theoretical way of confirming that a part is fit for purpose, and further provide a “best case” performance benchmark given exactly the right implementation.
Taking an EVM along when performing pre-compliance EMC tests is a quick way to confirm that the chosen part is not going to be an emissions nightmare in the final design, and similarly if the EVM overheats, your design probably will as well.
Lesson: Buy in an EVM for your chosen switcher part and perform a set of tests that represent the final use case. If you have problems at this stage, it’s fair to say you should pick a different part or modify your design to mitigate the issues.
Saturation current is not standardized
If your switcher’s primary inductor becomes saturated, the efficiency of the system will rapidly plummet, and it will likely lose regulation or overheat. Most switcher manufacturers helpfully include the necessary inductor current calculations to enable you to make the correct part choice; however, the inductor datasheets can themselves be misleading.
Saturation current is universally defined as the current at which inductance decreases by a certain percentage, but different manufacturers use different percentages. Wurth often take a 20% inductance drop to mean saturation, but other manufacturers may use a 50% or even 80% drop.
Careful consideration must be given to peak inductor current, not just expected output current. High efficiency switcher designs typically look to minimize switching time and thereby losses, but this inherently drives up peak inductor current (and also EMC emissions!)
Lesson: When specifying an inductor, check what inductance drop the saturation current parameter refers to. If 80%, then you need to design more current headroom into your system to maintain correct regulation. Pay attention to peak currents!
Switchers aren’t always best
Switchers are more efficient than linear regulators, so does that mean they should be used wherever possible? Not quite.
Look at the efficiency curves on the datasheet. See that bit where the efficiency curve falls off a cliff at low loads? If your mobile widget, for example, needs a 3V0 rail derived from a 3V7 LiPo, and spends most of its time in a micro-power sleep mode, your switcher will likely burn more power than an LDO in those periods when the load is not drawing much current. If you then add in inductor switching losses and quiescent current, you could end up throwing a lot more power away through trying to use an “efficient” switcher than by using an “inefficient” linear regulator.
Lesson: When designing power supplies for very low power devices, consider if a switcher is honestly the best option. Even low power optimized designs (such as Analog Devices Micropower ICs) may still yield worse results overall than a carefully specified LDO in a low power application, so a system level power budget analysis is a must.
Keep it (the high current path) simple
Switchers create circulating paths of rapidly switching high currents as part of normal operation. If you don’t carefully implement these paths, then the system is likely to radiate or couple excess noise to other parts of the system, causing yet more EMC issues.
If possible, design the converter on a single layer to avoid layer transitions by vias, and with short, low impedance paths between the diode, inductor, and capacitors on the output side, with similarly low impedance paths between the input capacitors and switch FET. The ground path between all these components should also be robust and low impedance. If using a four-layer board, placing a ground plane under the switcher can suppress coupling to other parts of the circuit, but make sure to follow layout guidelines for the particular part, as coupling between traces and ground can be relevant to operation and performance!
Lesson: Keep the entire switcher design compact, and keep the ground return path short and on the same layer if possible. Ground planes and careful splits can be useful for reducing coupling of the circulating currents into other parts of the system.
This is far from an exhaustive list of all possible design considerations for DC to DC converters, but hopefully it gives you some useful guidelines on what to look out for when embarking on a new power supply design, particularly for those at the start of their engineering career.
Here at CDP we employ a talented range of engineers across all disciplines who solve problems like these on a daily basis. If you are interested in joining our team, check out our vacancies in Cambridge, UK or Raleigh, N.C. USA.
If on the other hand you are experiencing these sorts of issues at your company, please do reach out to CDP to see how our business can help yours.
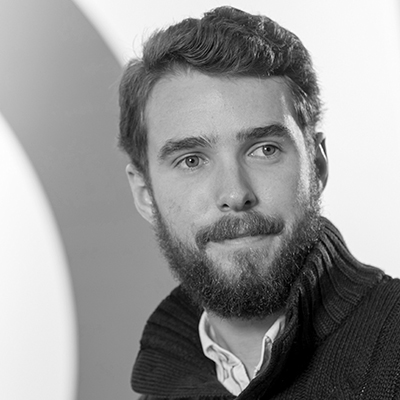
Finlay Knops-Mckim
Consultant Electronics Engineer