Innovations in Oncology: Past, Present, Future
on LinkedIn:
This article will explore five exciting developments in the field of oncology and how advances in diagnostics, data analytics, cell biology and delivery devices are enabling oncology companies to bring the hope of longer, healthier lives to a wider range of patients than ever before.
First, let’s look at the history of this field of medicine and the current state of play. It is a disease that has plagued us for millennia. The oldest known records date as far back as Ancient Egypt, when the great physician Imhotep described a “bulging in the breast” for which there was no therapy. Thankfully, we’ve come a long way since.
A history of oncology: a whistle-stop tour [1]
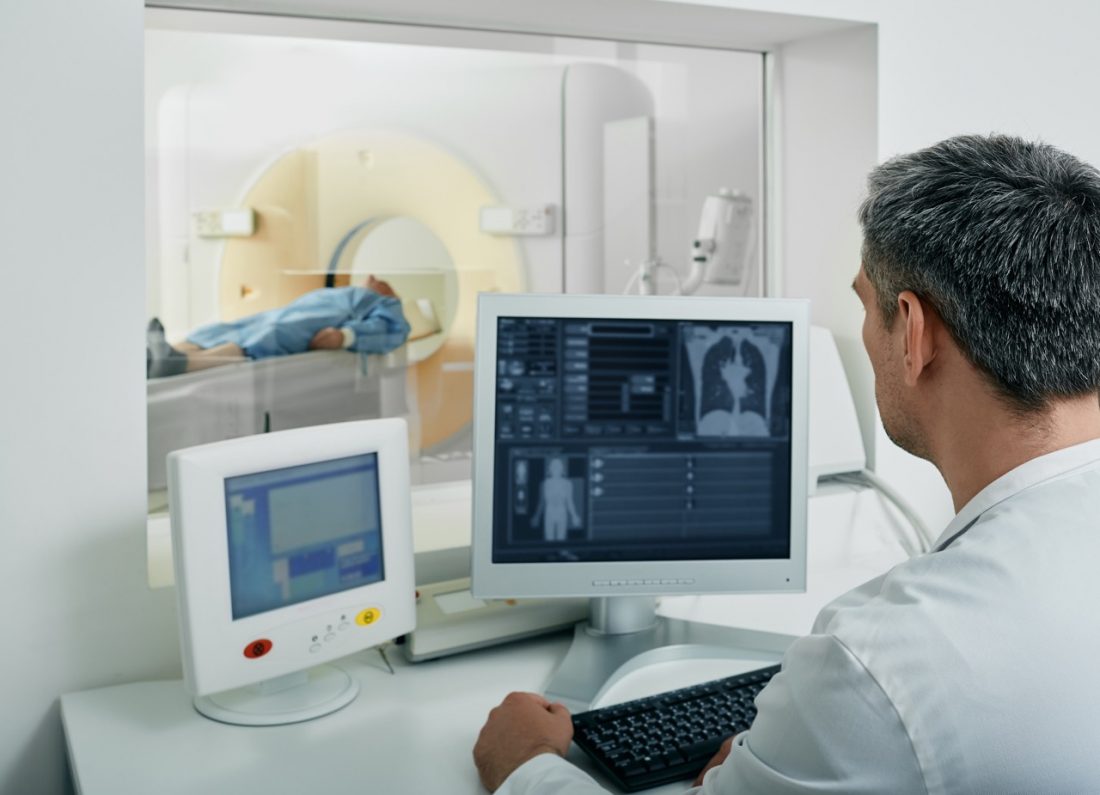
Modern surgery (the 1800s):
Surgeons faced severe limitations until the discovery of anaesthesia and antisepsis in the late 1800s. By the early 1900s, sophisticated surgeries had become commonplace, and it was possible to treat a wide range of early-stage cancers by surgically removing solid tumours.
Radiation and chemotherapy (late 1800s-1900s):
The discovery of X-rays in 1895 ushered in the era of radiation therapy. Chemotherapy followed in the mid-1900s, with the first effective cytotoxic drug found in nitrogen mustard, a derivative of the mustard gas used as a chemical weapon in WWI.
Progress in the 1950s-60s:
A flurry of discoveries in the 1950s and 60s yielded gains in cancer prevention, detection, and treatment. Notable achievements include proving the link between smoking and lung cancer, the introduction of cervical cancer screening, and advancements in chemotherapy.
The knowledge revolution (1980s-present):
The 1980s marked a turning point in our understanding of cancer biology. What was once thought of as a monolithic disease, and then a collection of altogether disparate conditions, is now understood to be a group of related diseases. Underneath the heterogeneity of cancer lies a consistent pattern: mutated genes that typically sit at key junctions in cellular signalling pathways, granting the cell distinctive pathological capabilities (e.g., the ability to evade growth-curbing mechanisms).
With the discovery of proto-oncogenes, tumour suppressor genes, and an understanding of the ways in which they disrupt specific cellular pathways, came the promise of (molecular) targeted therapies. Herceptin was one of the first: a monoclonal antibody specifically engineered to target and block receptors encoded by the HER2 oncogene.
New insights also paved the way for better prevention and diagnostics, such as the development of preventative cancer vaccines and the use of cancer biomarker assays to improve clinical decision-making.
Oncology today: a global challenge
The knowledge revolution continues, with over 700 proto-oncogenes and tumour suppressor genes identified by 2018, providing a cornucopia of potential treatment targets. However, the focus has shifted from “finding a cure” to prolonging healthy life through better prevention and care. And it’s working: in the US, the age-adjusted cancer death rate dropped by 22% between 2005 and 2020 [2]. But challenges persist, including:
- Detecting cancer early when it can be most successfully treated
- Identifying the best treatment for each patient
- Targeting treatments to kill cancer cells while minimising off-target toxicity effectively
- Increasing equitable access to prevention and care
Oncology tomorrow: a multidisciplinary solution
Opportunities that were previously unimaginable are now within our grasp – we have unprecedented insight into cancer and access to powerful new technologies. Here are five developments we’re excited about:
1. Investments in decentralised testing
In the world of oncology diagnostics, decentralised testing can help more patients get an earlier and more accurate diagnosis, improving their chances of survival.
Elsewhere in diagnostics – notably respiratory illnesses and sexually transmitted diseases – there has been a successful decentralisation of testing. An abundance of point-of-care tests are available, along with the infrastructure to support them. By speeding up diagnosis, these tests streamline the process from the appearance of symptoms to the patient receiving the correct medication.
In cancer diagnostics, this decentralisation hasn’t been possible to date. The vast majority of testing still requires a biopsy and subsequent analysis in a pathology lab; throughput is therefore constrained.
Ultimately, science is only part of the answer.
There is also a need to improve workflows across
the entire diagnostic journey, from sample collection
and preparation to data interpretation.
Emerging cell-free DNA methods, which use liquid biopsies, can potentially reduce the reliance on solid tumour biopsies, but limited sensitivity restricts their applicability. Ultimately, science is only part of the answer. There is also a need to improve workflows across the entire diagnostic journey, from sample collection and preparation to data interpretation. Here, too, progress is being made. For example, the UK has seen investment in Community Diagnostic Centres – vastly increasing computed tomography (CT), magnetic resonance imaging (MRI), and ultrasound scanning capacity to reduce the time to diagnosis [3].
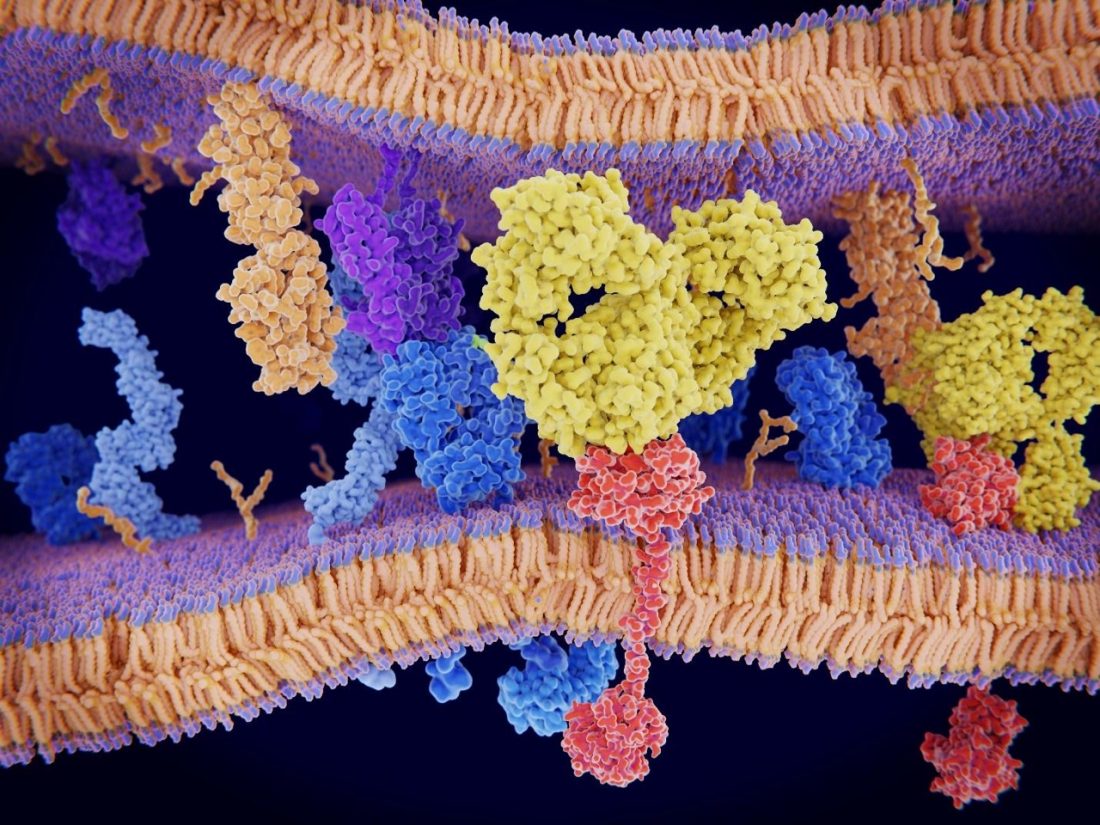
2. Big data meets precision oncology
Once a diagnosis is made, clinicians must choose the most appropriate course of treatment. High-throughput sequencing technology and large-scale cancer genome studies have put a wealth of data at their disposal to aid in this decision. Precision oncology aims to harness that data to improve outcomes by using mutation analysis to guide treatment decisions for individual patients. However, making this a reality has turned out to be far more complicated than it sounds.
To be clinically useful, genetic or biomarker tests should be sufficiently predictive of treatment response from a targeted agent (e.g., HER2 positivity in breast cancer is predictive of susceptibility to Herceptin). Finding such test/treatment targets in the vast quantities of multi-omic data by brute force (and then developing or pairing with suitably matching drugs) requires immense computational power, which has so far been a limiting factor.
Because of its superior pattern recognition capabilities,
we’re excited about the potential for artificial intelligence (AI)
to change the game entirely.
Because of its superior pattern recognition capabilities, we’re excited about the potential for artificial intelligence (AI) to change the game entirely. For example, AI could leverage the ever-growing stores of data to more efficiently:
- Identify driver mutations that may also be actionable drug targets
- Generate and down-select potential drug candidates with in-silico screening
- Identify predictive biomarkers that could be used to match patients with the most appropriate therapy
Of course, it’s early days for the application of AI for this purpose and making the best use of the data requires as much of it as possible to be publicly accessible, as well as the development of standard tools and conventions to improve cross-centre collaboration – so we’ll monitor developments with interest.
3. Foundational models of the cell
The promise of AI in oncology goes well beyond pattern recognition: just as foundation models have been developed for language (and are now used to power chatbots and generative AI), they could be developed for biological systems. Imagine general-purpose models of human cells of human cancers that could be adapted to represent specific patient cohorts or even individual patients (e.g., digital twins).
The models might be quite intricate, representing
a hierarchy of structure – from organ systems,
through tumours and their microenvironments,
to individual genes and the proteins they encode.
The models might be quite intricate, representing a hierarchy of structure – from organ systems, through tumours and their microenvironments, to individual genes and the proteins they encode. They could be adapted to assist in drug discovery, trial design and monitoring, and clinical decision-making. For example, to:
- Simulate the effect of potential treatments on different patient populations
- Evaluate treatment options in a specific cohort
Key to the successful implementation of such models is explainability: the ability to explain the model’s behaviour and its decisions in human terms. Additionally, to unleash their full potential, these models should not be static but rather learn continuously as new data is acquired – which will pose an interesting regulatory challenge.
4. The right device for the right patient at the right time
Once an appropriate therapeutic agent has been identified comes the challenge of delivering it safely and effectively. Here, the choice of administration route and delivery device are crucial.
In therapeutic areas such as rheumatoid arthritis, drugs that were previously delivered intravenously (IV) have long been available for subcutaneous (SubQ) self-administration – a transition made possible by improvements in formulation as well as device technology (e.g., autoinjectors).
A similar trend of IV to SubQ for in-home self-injection has been forecast for anticancer drugs for some time, with the promise of reducing the treatment burden for patients and healthcare systems [4]. It hasn’t quite materialised, and for good reason:
- Most traditional cancer therapies are vesicant (i.e., known to damage subcutaneous tissue) and, therefore, fundamentally unsuitable for SubQ administration
- Many are hazardous to handle: the risk of a leakage causing harm is high in a home or self-administration setting
- Many patients need to be seen face-to-face by a clinician because their therapy requires variable dosing or close monitoring for side-effects
… there is certainly room for subcutaneous
delivery in oncology and even self-administration
– if the risk/benefit profile for a specific
patient and therapy warrants it.
As evidenced by the small but growing number of regulatory approvals (mostly in the form of pre-filled syringes or syringes prepared by the hospital pharmacy), there is certainly room for subcutaneous delivery in oncology and even self-administration – if the risk/benefit profile for a specific patient and therapy warrants it.
The solution, however, is not a push for wider adoption of any specific device technology but rather device selection that is underpinned by a thorough understanding of all stakeholder needs. Not just the immediate needs of the patient, clinicians, and formulation but also the wider context in which the therapy will be administered (care workflow, reimbursement pathways, etc.).
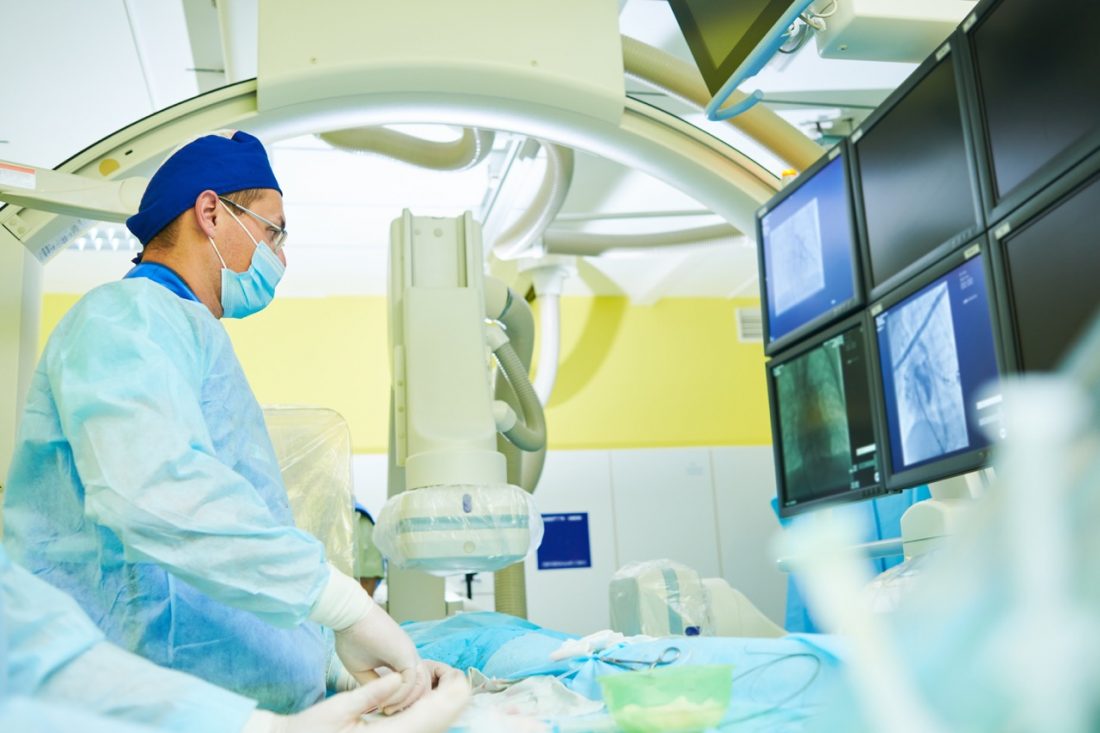
5. Devices for targeted drug delivery
Cytotoxic chemotherapy has long been the mainstay of oncology treatment: it is versatile and effective. The problem is that off-target toxicity limits its tolerability. Targeted delivery aims to address this challenge by increasing drug concentration in cancerous tissues relative to healthy ones. This can improve efficacy while reducing side effects.
Drug-loaded nanoparticles, which are delivered systemically, have been investigated since the ‘90s as a way to achieve selective binding to tumour targets. However, despite extensive research, the number of such therapies available to patients is well below projections; promise in animal studies often fails to translate to success in humans [5].
We’re encouraged by the potential of targeted
delivery, for example, direct injection of cancer
drugs into tumours, as well as the use of
implantable pumps and reservoirs.
We’re excited about the potential of targeted delivery, for example, direct injection of cancer drugs into tumours, as well as the use of implantable pumps and reservoirs to access pharmacological sanctuaries such as the blood-brain barrier.
There is a robust pipeline of intratumoral therapies, with drugs for melanoma leading the charge – partly because the lesions are often superficial and, therefore, easier to find and inject into. Delivery to deeper tumours is more challenging and is currently conducted by highly skilled clinicians with imaging support – therefore requiring the development of delivery devices that provide a high degree of flexibility and control over the injection technique
Early diagnosis and targeted treatment offer hope of better outcomes
Oncology has come a long way and will continue to evolve with our growing understanding of the disease and the emergence of new technologies to prevent, detect and treat it. We’re excited to see innovations along the entire care pathway:
- Investments in decentralised testing, including the development of cell-free DNA technologies, to allow faster and more accurate diagnosis
- The potential of AI to revolutionise drug discovery and development and to help clinicians match patients to the most appropriate treatment
- Improvements in delivery device technology to allow for safer, more targeted, and effective treatment
The journey continues, and hope prevails.
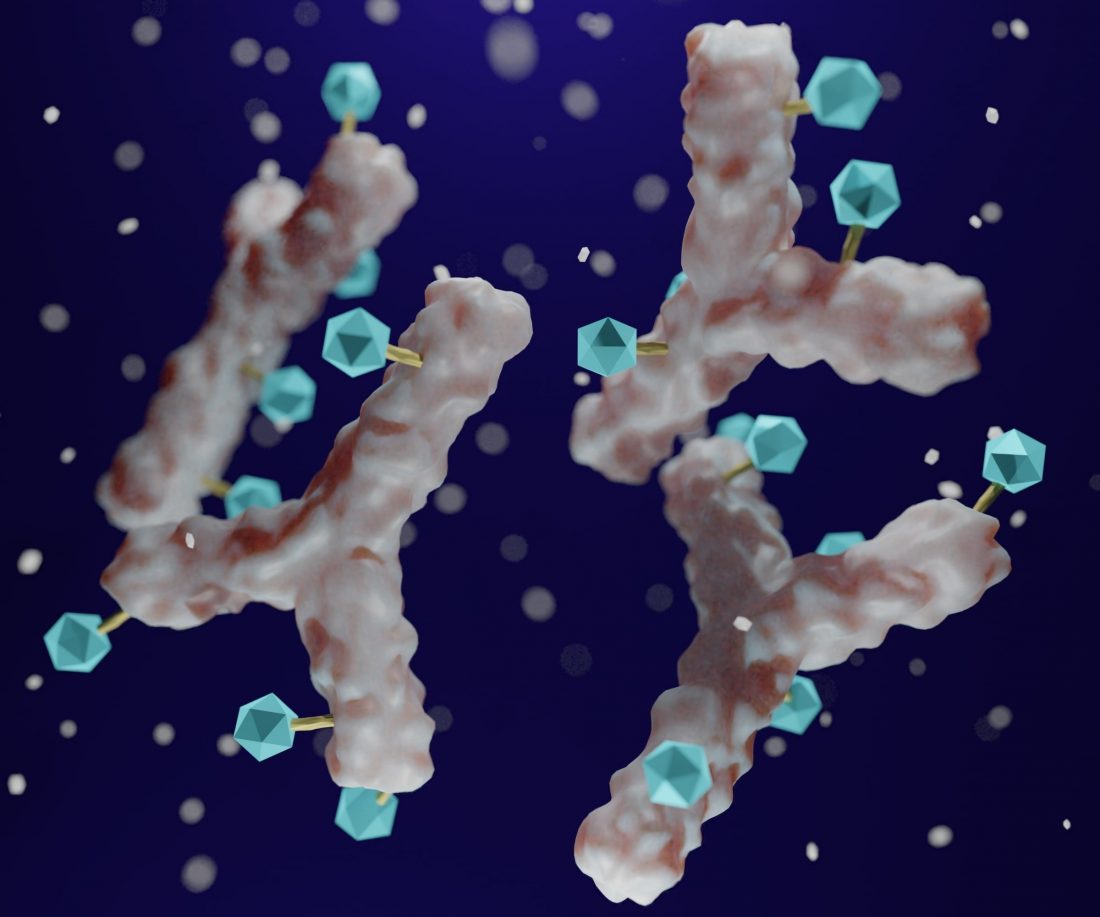
References
- The Emperor of All Maladies: A Biography of Cancer, by Siddhartha Mukherjee
- National Center for Chronic Disease Prevention and Health Promotion (U.S.). Division of Cancer Prevention and Control. An Update on cancer deaths in the United States (2022).
- https://www.gov.uk/government/news/government-to-deliver-160-community-diagnostic-centres-a-year-early
- Levêque, D. Subcutaneous Administration of Anticancer Agents. Anticancer Research 34 (4) 1579-1586 (2014).
- Mitchell, M.J., Billingsley, M.M., Haley, R.M. et al. Engineering precision nanoparticles for drug delivery. Nat Rev Drug Discov 20, 101–124 (2021).
Our capabilities
See our range of capabilities in developing drug delivery devices, diagnostics, and medical therapy solutions by visiting the below pages.

María FM Balson
Consultant Biomedical Engineer