Our brains in space: The next giant leap
on LinkedIn:
Fifty-two years after the first lunar landing, NASA’s program Artemis prepares to land on the moon. It’s a major step towards future space explorations and Mars missions – one that’ll see women in deep space for the first time.
The technology being developed includes the next generation of rockets, space suits, lunar landers, and gateways for travel into deep space. However, we’re yet to solve one of the most fundamental aspects of long-duration space travel: how to keep our brains alive.
The human brain is a remarkable feat of physiological engineering. It has sensing systems, self-regulating mechanisms, valves, dampening features, adaptable form, and even a set of complex networks that developers of the most advanced AI can only dream of. Yet, we still know surprisingly little about the interactions and boundaries of these systems and how they respond to space travel.
In this article, I explore how mathematical modeling is improving our understanding of the brain, successfully predicting the existence of anatomical features no one’s identified before – with learnings for Mars missions and the diagnosis and treatment of conditions such as Alzheimer’s disease.
What happens to our brains in space?
The human body is adapted to operate with Earth’s gravity. In space, microgravity (reduced gravity) causes fluids in the body to shift. This is most noticeable in the brain, where microgravity increases fluid volume, putting pressure on the nervous tissue.
Having a rigid cranium is good news for many reasons, but holding excess fluid isn’t one of them. Luckily, the increased intracranial pressure isn’t high enough to be immediately fatal. Still, it can introduce chronic knock-on effects with long-term implications.
Immediate brain-related effects experienced by astronauts due to increased intracranial pressure and fluid movement in the inner ear include headaches, dizziness, nausea, temporary blurred vision, and disturbed perception and spatial awareness. During space flight, the brain’s vestibular (orientation) system adapts to the new environment, reducing some of the effects. But others persist or appear in different forms, such as sleep disturbances.
After returning to Earth, astronauts who’ve taken long-duration flights have had one or more of these effects:
- visual impairment – ophthalmic changes due to excess pressure in the optic nerve
- brain ‘swelling’ – changes in ventricles (fluid-filled cavities) and grey and white matter (brain tissue composed of nerve fibers and nerve cells)
- pituitary gland shrinkage – downsizing of the hormone-secreting gland that helps regulate body functions, including blood pressure and kidney function.
There’s a correlation between longer-duration space flight and increased severity of post-flight effects. These effects can be present long after the mission ends, and some can cause irreversible damage, for example, visual impairment.
Why does this happen?
The brain is protected for changes in gravity by a surrounding fluid called cerebrospinal fluid (CSF), a non-permeable layer called dura mater where CSF is contained, and one-way valves called arachnoid villi on the dura mater where CSF exits.
CSF is responsible for compensating changes in pressure, such as those created by the arteries or changes in posture. It’s also involved in cellular waste removal from the brain during sleep, ‘cushioning’ the brain to prevent trauma, and maintaining the best possible environment for the brain, for example, optimizing ion concentration levels to prevent cell death.
Dura mater is the ‘sac’ that contains the CSF. It plays a pivotal role in ensuring CSF exits the cranial and spinal spaces in a controlled way through the arachnoid villi.
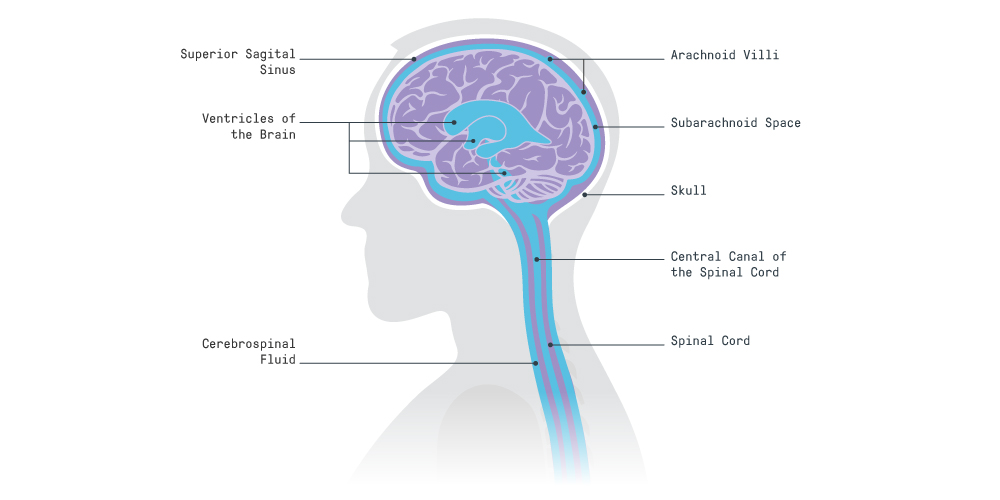
As gravity lessens, important events take place which exert pressure in the brain:
- CSF in the cranium is no longer free to flow towards the spinal spaces. It concentrates mainly in the cranial space, increasing intracranial pressure.
- The spinal dura mater, usually relaxed and undisturbed, becomes compressed by other fluids and nearby organs as they shift upwards.
- Upward movement of the brain and spinal cord stem obstructs one of the main CSF exit routes, the superior sagittal sinus (SSS, composed of arachnoid villi). This prevents relief from increasing CSF pressure in the brain.
Gravity influences low-pressure systems most, so blood flow in the veins is affected, causing ‘pooling’ where blood accumulates because of poor circulation. This matters because cerebral veins lack valves, relying on CSF to ‘push’ venous blood forwards. There’s also movement of organs in the upper body and extracellular fluid, putting extra pressure on the spinal cavity and the veins in the head and neck. Other effects play a role in what’s known as ‘fluid shift’. These include osmotic pressure changes (fluid motion between areas of different solute concentration levels), variation in heart rate and respiration, and changes to lymphatic dynamics (the movement of a fluid, known as lymph, from tissues and organs).
How do we model the brain?
Scientific efforts focus on three approaches:
- microgravity testing – space mission and Zero-G flights with, for example, lower-body negative-pressure gravity suits, which aim to ‘pull’ fluid away from the upper body
- on-Earth simulated testing – bed-tilt tests to measure physiological changes during bed rest
- mathematical modeling.
Given the promise of mathematical modeling in predicting difficult-to-measure behaviors, such as epidemiological events like the ongoing COVID-19 pandemic, it’s worth exploring further.
Modeling the brain and spinal cord in microgravity
As part of my research, I’m investigating the relationship between pressure fluctuations in the brain and spinal cord and their impact under different gravitational conditions.
Using mathematical modeling, I outline the relationship of a single arterial root vessel and its blood flow with the next generation of vessels. This root vessel branches into two vessels, and each of those to another two, and so on. Each generation carries information of the preceding vessel from which the rest of the parameters can be derived. The arterial branching continues until reaching the capillaries (tiny vessels where cellular nutrients and waste are exchanged), before converging again to complete the venous tree.
After the blood vessels are described, I add the CSF spaces in the cranium and spine. These spaces allow CSF to interact with the blood vessels, which I can study for behavior under different physiological and gravitational conditions.
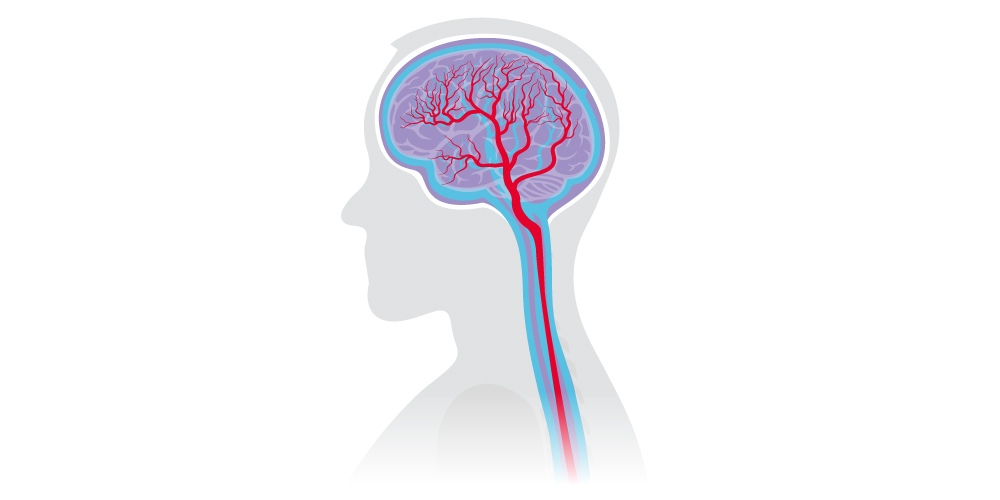
The model accounts for several key features of cerebral circulation, including autoregulation (the ability of blood vessels to expand and contract to metabolic demands and pressure changes), CSF exit routes (arachnoid villi), and gravitational components. As I add gravity to the model, I can test different postures, for example, lying flat on the back (supine).
By mathematical modeling, I successfully predicted the existence of anatomical features that had never before been identified – arachnoid villi in the spinal dura mater. These additional exit routes appear to be a crucial element to relieve CSF pressure under different gravitational conditions. The model showed that the cranial and spinal exit routes work together to keep CSF pressure fluctuations stable, ensuring undisturbed cerebral blood flow.
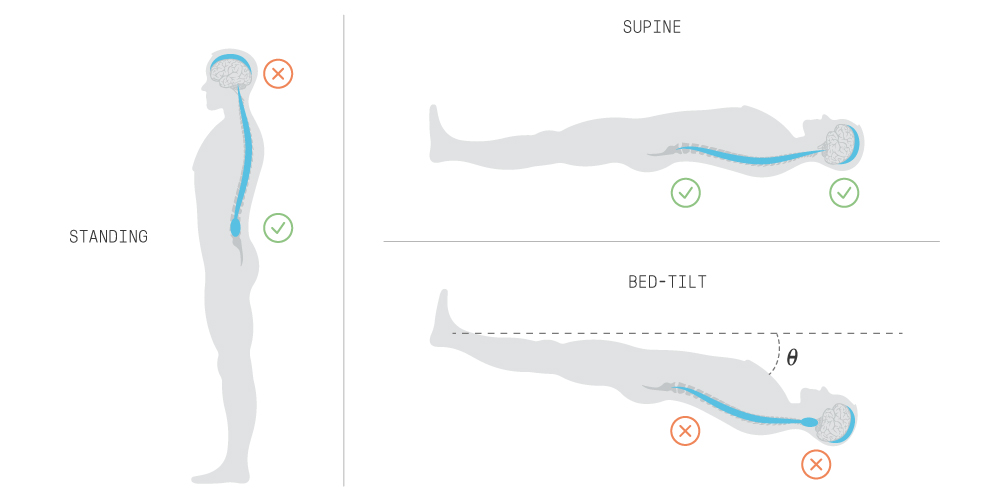
On Earth, standing allows CSF to shift downwards, lowering pressure inside the cranium, which causes the cranial exit route to close. The spinal exit route can work as the main exit route, but it also has to deal with increased pressure outside the spinal cavity, such as the interstitial fluid (fluid between cells) of surrounding organs. If the external pressure is greater, the spinal exit route closes, and the pressure inside the cranium increases until the cranial exit route opens again. A supine position allows exit through either the cranial or spinal routes.
In microgravity, the upward shift of fluid causes CSF pressure in the cranium to increase, whether standing or supine. This can be modeled using a bed-tilt test where the body is positioned at a negative angle. The spinal exit route closure intensifies CSF intracranial pressure because of increased pressure from other fluids and organs on the spinal cavity. The combined effect forces the cranial exit route to open to stabilize pressure fluctuations. However, recent research suggests the upward movement of the nervous tissue (brain and spinal cord) may block the main cranial exit route (the SSS). Blocking the SSS in the model leads to sustained intracranial pressure, resulting in irreversible consequences, which we see in space missions.
I also found that cerebral autoregulation plays a key role in overcoming gravitational changes in space and on Earth. Where autoregulation is absent or abnormal, constant blood isn’t delivered adequately to the brain, compromising oxygen to the brain. This can cause a range of conditions, including hypoxic events, where lack of oxygen leads to impaired or permanent nervous tissue damage.
All of this means that the brain, on its own, isn’t sufficiently adaptable to prevent tissue damage in microgravity environments. Sustained high intracranial pressure needs a path of least resistance to relieve the brain tissue of excess fluid. Extending space missions without fully understanding this behavior poses a significant risk.
The next stage of my modeling involves testing acute postural changes in combination with chronic physiological conditions, such as aging, to further analyze the effects I observed.
Why does this matter – and what can we do about it?
Beyond space exploration, understanding fluid movements in the brain and spinal cord is critically important to better diagnose and treat conditions such as Alzheimer’s disease, hydrocephalus (a life-changing and often fatal condition due to excess of CSF volume), spinal syringomyelia (a fluid-filled cyst disorder in the spine), stroke, and Multiple Sclerosis. Until we understand how these mechanisms can cause permanent damage, it’s vital to keep analyzing abnormal CSF-cerebrovascular behaviors under any gravitational conditions.
On whether we can go to Mars, it’s possible, but landing on Mars (roughly seven months of space travel each way) would increase the risk of developing long-term health conditions, and as the mission extends, so does the likelihood and seriousness of those risks.
However, by pursuing an understanding of those risks, we’ll understand our physiology better. What adaptations could be expected of the human brain under any gravitational condition? What can we learn from other mammals overcoming significant gravitational challenges, such as bats and giraffes? Can an understanding of human brain adaptations help us treat neurological or neurodegenerative conditions?
As we aim for ever more challenging space missions, mathematical modeling can help us characterize the boundaries of human physiology – and take the next giant leap in understanding our brains.
References
- Kramer, L.A., Hasan, K.M., Stenger, M.B., Sargsyan, A., Laurie, S.S., Otto, C., Ploutz-Snyder, R.J., Marshall-Goebel, K., Riascos, R.F. and Macias, B.R., 2020. Intracranial effects of microgravity: a prospective longitudinal MRI study. Radiology, 295(3), pp.640-648.
- Li, K., Guo, X., Jin, Z., Ouyang, X., Zeng, Y., Feng, J., Wang, Y., Yao, L. and Ma, L., 2015. Effect of simulated microgravity on human brain gray matter and white matter–evidence from MRI. PloS one, 10(8), p.e0135835.
- Petersen, L.G., Lawley, J.S., Lilja‐Cyron, A., Petersen, J.C., Howden, E.J., Sarma, S., Cornwell 3rd, W.K., Zhang, R., Whitworth, L.A., Williams, M.A. and Juhler, M., 2019. Lower body negative pressure to safely reduce intracranial pressure. The Journal of physiology, 597(1), pp.237-248.
- Roberts, D.R., Albrecht, M.H., Collins, H.R., Asemani, D., Chatterjee, A.R., Spampinato, M.V., Zhu, X., Chimowitz, M.I. and Antonucci, M.U., 2017. Effects of spaceflight on astronaut brain structure as indicated on MRI. New England Journal of Medicine, 377(18), pp.1746-1753.
- Roberts, D.R., Asemani, D., Nietert, P.J., Eckert, M.A., Inglesby, D.C., Bloomberg, J.J., George, M.S. and Brown, T.R., 2019. Prolonged microgravity affects human brain structure and function. American Journal of Neuroradiology, 40(11), pp.1878-1885.
- Sanchez-Cazares, K E 2018, ‘Mathematical Model of the Cerebral Circulation and Distribution of Cerebrospinal Fluid’, PhD thesis, Imperial College, London.
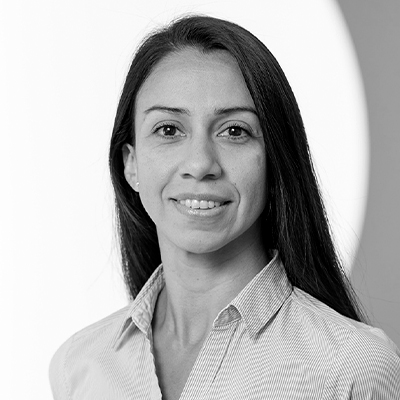
Karla Sanchez
Senior Consultant Biomedical Engineer